Wednesday was October 21st, Alfred Nobel’s birthday. In honour of the occasion and the recent announcements of the Nobel Prizes, I wanted to summarize some details surrounding the 2015 Nobel Prize for Physics which went to Dr. Takaaki Kajita and Dr. Arthur B. McDonald. The prize was awarded for their discoveries about the nature of sub-atomic particles called neutrinos.
This topic is particularly dear to me because, in 2013 I had to opportunity to visit SNOLAB where Dr. McDonald did his ground-breaking research.
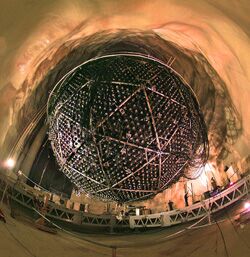
The Sudbury Neutrino Observatory
What is SNOLAB?
SNOLAB is a physics laboratory built around the original Sudbury Neutrino Observatory (SNO). As you can tell from the name, it’s located in Sudbury, ON. The lab doesn’t offer public tours, but they will provide tours for school/education groups.
While I was a graduate student in Laurentian University’s Science Communication program, I got a chance to visit the facility. Samantha Kuula, SNOLAB’s Communications Officer, is a graduate of the Sci Comm program and was able to give us a tour. A few days before the field trip, Samantha paid the class a visit to go over the protocols and what we should expect. It was an extensive presentation.
The morning of the tour we had a super early start and it took about half an hour to drive from the city to SNOLAB’s above-ground facility at Vale Creighton Mine. There, we donned mining gear: hard hats with headlamps and safety headphones, work gloves, safety belts, reflective coveralls and big’ol rubber safety boots.
The elevator to the lab only went down at scheduled times and you did not want to miss it. We all packed in with other folks headed to the lab and the mines. SNOLab is part of an active mine. The elevator was dark and crowded and chilly. My ears popped on the way down. That’s to be expected when you travel 6,800 feet blow ground. We disembarked and took a short headlamp-illuminated hike from the elevator to the actual facility.
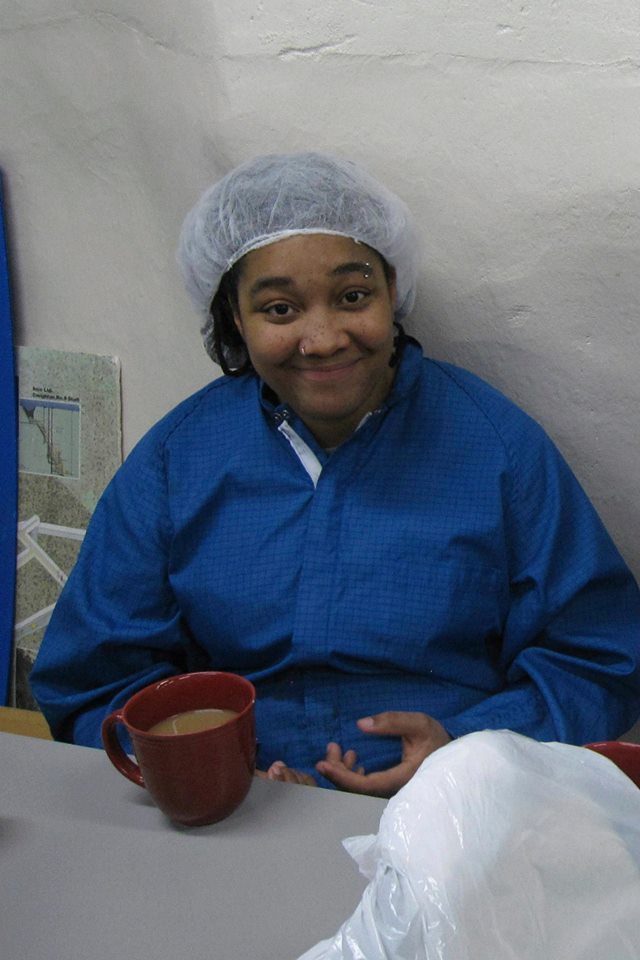
It’s not as impressive as SNO, but this is evidence that I had coffee at a leading-edge research facility.
But before we could enter the lab we had to shower. That hike through the mine drift left us covered in dust. We left our gear and clothing in the locker area scrubbed and washed our hair and put on ‘clean’ suits with hairnets before we could even enter the employee area.
Once we had a break in the coffee room, the tour began. I regretted not bringing my camera or even my phone. The experimental apparatuses, while inspiring in their purposes, were also gorgeous. Mirrors, reflective metal and great big shiny spheres. Some were small enough to fit on a table, others were rooms, caverns. It was remarkable.
I didn’t leave my gear above ground because of forgetfulness, though. Not only did we have to get cleaned before we entered the lab, but any equipment brought down had to be cleaned as well and I was a little worried about it. All electronics had to go through the a separate cleaning system to get rid of any contaminants they might carry.
If mine dust is a problem, why have a physics lab in a mine?
The Sudbury Neutrino Observatory is unique: it is a science laboratory 2km underground. Most of the research focuses on sub-atomic particles (particles smaller than atoms) and astronomical particle physics.
It’s ironic to have an ‘observatory’ below-ground. When people hear the word, they may think of telescopes on top of hills. But being underground is necessary for the kind of observations scientists are trying to make. The surface of the Earth is bombarded in cosmic radiation constantly. It is nearly impossible for researchers to isolate the particles or signals they’re interested in with all that ‘noise’.
The 2 km of rock above the lab acts as natural shielding for the experiments. The rock filters out larger, more reactive particles and allows detectors to pick up what the researchers are interested in. So after using a really thick rock ceiling to protect the experiments, the last thing the scientists want is for someone to track mine dirt into the lab that may contain particles that could stimulate their detectors.
As the name suggests, neutrinos are one of the hard-to-detect particles studied at SNOLab.
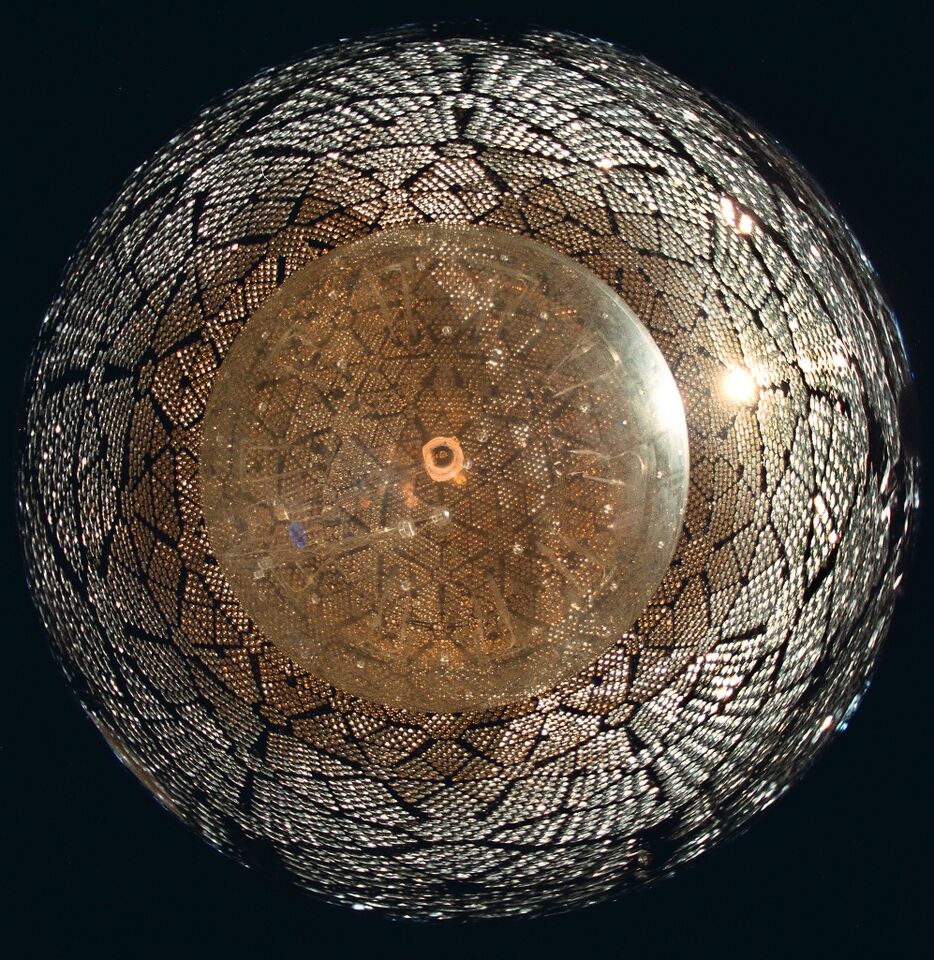
The inside of the SNO detector
What are neutrinos?
Neutrinos are sub-atomic particles (i.e. smaller than atoms) traveling near the speed of light. They are non-reactive, electrically neutral (neither positively charged nor negatively charged), and until recently they were believed to have no mass. Neutrinos are numerous, the only particle that outnumbers them are photons (light particles). Neutrinos come in three different flavours: electron-neutrinos, muon-neutrinos and tau-neutrinos. Yes, ‘flavour’ is the technical name and no neutrinos are not tasty.
In fact, they’re so small and react so infrequently with matter that millions of them pass through your body all the time and you never notice, much less taste them. Billions pass through Earth with no trouble at all. They come from reactions like natural and human-produced radioactive decay. Some are created when cosmic radiation collides with our atmosphere (producing muon-neutrinos). Others are created by reactions deep in the sun’s core (reactions that produce electron-neutrinos).
So, what did Dr. Takaaki Kajita and Dr. Arthur B. McDonald discover?
Dr. Kajita conducted his research at the Super-Kamiokande Detector in Japan. There, he measured electron-neutrinos and muon-neutrinos. Remember that:
- Muon-neutrinos are formed by cosmic radiation reacting with our atmosphere
- Neutrinos can pass through the entire Earth without hitting anything
Dr. Kajita made observations of the muon-neutrinos that showered directly from the atmosphere above Japan and the muon-neutrinos formed halfway around the world that then passed through the Earth to Super-Kamiokande.
The research team expected there to be the same number of muon-neutrinos hitting the detector from above and below. However, muon-neutrinos showering over Japan were detected more often than muon-neutrinos that passed through the Earth and were supposed to hit Super-Kamiokande from below.
The values for the electron-neutrinos were in line with the researchers’ expectations. Something was happening to the muon-neutrinos on their journey through the globe. Unfortunately, Super-Kamiokande could not detect tau-neutrinos and so the possibility that muon-neutrinos could turn into tau-neutrinos remained speculation.
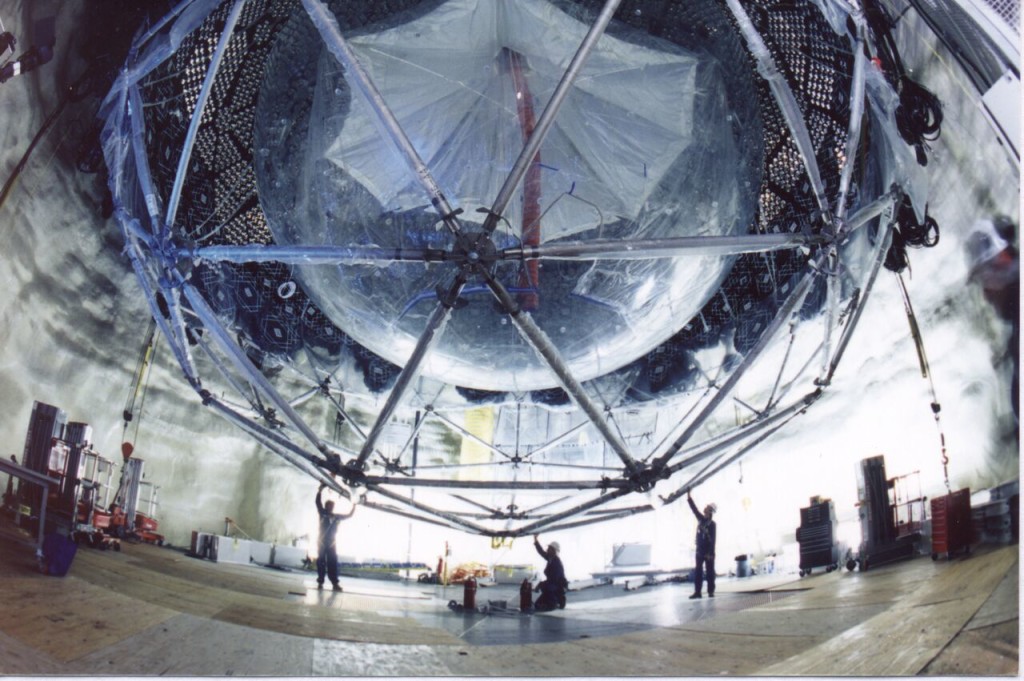
SNO under construction
An answer to this question came from research out of SNO lead by Dr. Arthur McDonald. Dr. McDonald’s experiments focused on electron-neutrinos generated by the Sun. The detector was able to monitor two kinds of reactions:
- Reactions produced by electron-neutrinos
- Reactions produced by all three flavours without distinguishing between them
For the first kind of reaction, the researchers could say, “That was an electron-neutrino”. For the second kind of reaction the researchers could say, “That was an electron-neutrino, or a muon-neutrino, or a tau-neutrino…It was a neutrino, ok.”
This ability to detect all three types is really important to teasing apart Dr. Kajita’s problem of missing muon-neutrinos. The SNO researchers knew how many electron-neutrinos they expected and if this number was much lower than the total number of neutrinos then something was happening to the electron-neutrinos.
SNO began making observations in 1999. It detected fewer electron-neutrinos that expected. But the total number of neutrinos (electron-, muon-, and tau-) matched what the researchers expected. This was evidence that electron-neutrinos produced by the Sun were changing into either muon- or tau-neutrinos on their long journey to Earth.
What do these observations mean? Remember when I said that scientists used to think that neutrinos had no mass? Well, the fact that neutrinos can change their type means that they do have mass. This idea is huge! It pokes a hole in the current understanding of sub-atomic particles, how they exist and what they’re like. Hence, it deserves a Nobel Prize.
New research is being done at SNOLAB to study neutrinos. While on the tour, my classmates and I learned about a new project, SNO+. It will use SNO’s apparatus and structure but with some changes that will allow researchers to study solar neutrinos with lower energy levels. By continuing this and other detection experiments, researchers can gain more information about these elusive particles.
If you’re interested in learning more about the Nobel Prize winning research, I recommend you check out this document released by the Nobel Prize Committee. It’s targeted at a general audience so it’s far easier to get through than the document targeted to a physics audience.
To end, I came across a short clip of Dr. McDonald “explaining” his research with the most Canadian analogy ever. Hope you enjoy.
Feel free to leave your comments below. I’d love to hear your feedback on this post.
Cheers,
Images
The images of SNO were generously provided by Samantha Kuula from SNOLAB.
That photo of me with coffe with generously provided by my much braver classmate who brought their camera down to SNOLAB.
Resources:
- “The Nobel Prize in Physics 2015”. Nobelprize.org. Nobel Media AB 2014. Web. 19 Oct 2015. <http://www.nobelprize.org/nobel_prizes/physics/laureates/2015/>
- “Takaaki Kajita – Facts”. Nobelprize.org. Nobel Media AB 2014. Web. 18 Oct 2015. <http://www.nobelprize.org/nobel_prizes/physics/laureates/2015/kajita-facts.html>
- “The Chameleons of Space” http://www.nobelprize.org/nobel_prizes/physics/laureates/2015/popular-physicsprize2015.pdf
- “Neutrinos” https://www.snolab.ca/sites/default/files/SNOLAB%20-%20Neutrinos.pdf
No Comments